Table of Contents
Introduction
Liver transplantation (LTx) is currently the most powerful treatment for end-stage liver disease. Benefitting from advances in surgical techniques, remarkable improvements in transplant recipient survival have been achieved in the last decades since Dr. Starzl conducted the first human LTx in 1963 (1). As an immunoregulatory organ, liver allograft in the transplant setting is more tolerogenic compared to other organs such as the kidney, heart, and intestine. It is reported that almost 20% of stable and carefully selected liver transplant recipients can be weaned safely off all immunosuppression (IS) (2). However, the majority of liver transplant recipients still require open-ended, or even lifelong IS to control the unwanted alloimmune responses, which is dominantly mediated by long-term, high magnitude CD8 T cells with the help of secondary lymph nodes and CD 4 T cells. Long-term or overdose IS treatment can lead to serious side effects such as severe infections and malignancy recurrence post transplantation (3–5). Therefore, understanding the mechanism of liver transplant tolerance and crosstalk between immune cells, especially alloreactive T cells and liver cells, can shed light on more specific tolerance induction strategies for clinical translation.
The liver receives 75% of the blood from the portal vein, which is rich in antigens and microbial products originated from the stomach, gut and spleen, and 25% of the blood is oxygenated from the hepatic artery (6). Thus, the hepatic immune system is tightly controlled and regulated under physiological conditions. In addition to the leukocytes from the blood flow through the liver, the liver itself consists of hepatocytes, hepatic stellate cells (HSCs), liver sinusoidal endothelial cells (LSECs), cholangiocytes, and a diverse array of immune cells residing within or trafficking to the liver (7). Crosstalk between liver cells and immune cells plays a central role in keeping the balance of immunity and tolerance. In general, innate immune cells such as dendritic cells (DC) and liver-resident DCs (Kupffer cells) serve as professional APCs to T cells, thereby mediating hepatic immunity. Interaction of innate immune cells and liver cells has been reviewed intensively by others (8–10). As alloreactive T cells or memory T cells mediated rejection represents a major hurdle to successful transplant tolerance induction, in this review, we mainly focus on the crosstalk between alloreactive T cells and liver cells in the transplant setting together with potential therapeutic prospects for tolerance induction.
T Cell Mediated Rejection With Alloantigen Recognition Pathways
When a liver is transplanted from the donor to the recipient, the alloantigen—mainly the allogenic major histocompatibility complex (MHC), or human leukocytes antigens (HLA) in humans—is ubiquitous, persists probably for life, and can be presented by both professional and unprofessional antigen presenting cells (APCs) at numerous sites. Thus, transplant rejection is mainly caused by the mismatch of MHCs or HLAs even in LTx. Alloantigen activated helper T cells (Th) secrete cytokines including TNFα, IFNγ, and IL-2 to further enhance the innate immune responses upon alloantigen challenge; on the other hand, they also stimulate effector CD4 T cells and cytotoxic CD8 T cells to express granzyme and perforin, thereby attacking the liver graft. In addition to the cell-mediated acute rejection, donor (graft) specific antibody (DSA) mediated humoral immune response is another important reason for hyper-acute rejection and chronic rejections. DSA mediated rejection is initiated by and in conjunction with T cell mediated alloimmunity (11–13). Several groups have shown that increased memory T cells or stem-like memory T cells correlate to allograft rejection or graft vs. host disease (GvHD) in human and animals. Stem-like memory T cells have the capacity to not only reconstitute the full diversity of memory and effector T cell population, but also maintain their own pool size through self-renewal (14–16). Therefore, memory T cells, especially donor-antigen specific memory T cells, are a major obstacle for successful tolerance induction. Moreover, as the counterpart of conventional T cells, regulatory T cells (Tregs), which are a specialized CD4 T cell subpopulation with the key transcription factor FoxP3 expression, are found to play an essential role in operational tolerance post solid organ transplantation. We showed previously that memory Tregs had superior capacity compared with naïve Tregs through higher expression of CD25 (IL-2 receptor α chain), CD39, CTLA-4 and other important molecules (17, 18). Nevertheless, the formation of immune memory initiate through alloantigen recognition and alloreactive T cells response is the backbone of adaptive immunity to allograft in the transplant setting (19). Notably, the alloimmune response is distinct from the immune response to classically pathogenic antigens because the alloreactive repertoire is highly diverse, especially in the naïve T cells subpopulation, as we showed before with next-generation sequencing (NGS) technology (18). The T cell receptor (TCR) provides a unique identity for each cell clone with around 2.5*107 TCRs for human naive T cells in each individual; the TCR repertoire against a given allogenic MHC haplotype is believed to be <10% of the entire TCR repertoire (19, 20). Therefore, recognition of the alloantigen is the first critical step for the following immune response or tolerance induction in the transplant setting.
To recognize the alloantigen by host TCRs, there are mainly 3 pathways: (i) direct way, (ii) indirect way, and (iii) semi-direct way through cross-dressing of graft MHC by host dendritic cells (DC) (19). Firstly, as shown in Figure 1A, through the direct recognition way, allograft APCs present the alloantigen with their own MHC-I molecules to the host CD8 T cells and allograft MHC-II to the host CD4 T cells. The intact antigen (protein) is recognized directly without the processing procedure. Direct recognition of the alloantigen is believed to be the dominant pathway of transplant rejection, which also includes the passenger leukocytes theory. “Passenger leukocytes” refer broadly to all the graft-derived immune cells that are transferred to the host secondary lymphoid tissue and trigger allograft rejection by direct recognition of the alloantigen (21–23). However, the contribution of passenger leukocytes to allograft rejection or tolerance induction is still not clearly understood. Irradiation of the allograft before surgery in rodent models results in killing of the graft lymphocytes and transplant rejection in otherwise tolerant recipients, suggesting the tolerance induction role of donor-derived graft-resident lymphocytes (24–26). On the other hand, the majority of donor lymphocytes are replaced by recipient bone marrow derived hematolymphoid cells within months post LTx (27–29). Nevertheless, direct recognition of the alloantigen by CD4 T cells was considered to persist at early time points after transplantation and was highly correlated with the lifespan of graft DCs (30). Whereas, indirect recognition of alloantigen is considered to be related with both acute and chronic transplant rejection. By this way, alloantigen is internalized and processed by host APCs into peptide antigens, which are further presented with host MHC molecules and thereby recognized by the TCR repertoire of host T cells (Figure 1B). CD4 T cells response from the indirect recognition way is believed to be more relevant with the allograft rejection than CD8 T cells in solid organ transplantation due to the relatively low expression of host MHC-I antigen epitopes in the vascularized allografts (31, 32). Last but not least, through the semi-direct recognition way, the host DCs acquire expression of the graft MHC molecule, which is also called cross-dressing of the host DCs, then re-present the graft MHC-antigen complex as intact alloantigen to the host T cells without further processing (Figure 1C). This phenomenon was also observed by Ono et al. (33) that in a mice LTx model, graft interstitial DCs decreased rapidly post LTx, then they were replaced by host DCs, which peaked at day 7 and persisted indefinitely. Around 60% of the host DCs in the liver graft expressed graft MHC-I, suggesting cross-dressing, and controlled the proliferation of anti-graft host T cells. On the other hand, non-cross-dressed DCs failed to suppress the anti-graft T cell response (34–38). The mechanism behind cross-dressing is believed to be related with cell-cell contact or extracellular exosomes (39–44).
Figure 1. Schematic illustration of three alloantigen recognition pathways. (A) Direct recognition of alloantigen: allograft antigen-presenting cells (APCs) present intact alloantigen directly to host T cells. (B) Indirect recognition of alloantigen: host APCs process and present the allograft-derived peptides to host T cells, mainly CD4 T cells. (C) Semi-direct recognition way: host APCs, mainly dendritic cells (DCs), acquire graft MHC molecules, which is called cross-dressing, and present the peptide directly to host T cells.
Through collaboration of different alloantigen recognition pathways, host CD4 T cells are activated by continued TCR stimulation with graft MHC-II alloantigen, which are expressed either on the surface of graft APCs or re-presented by host DCs through semi-direct recognition within secondary lymphoid tissue. The principle role of the indirect pathway in CD4 T cell response, which mainly focuses on self-restricted, processed alloantigen, is likely at the late phase of transplant rejection through providing help for cytotoxic T cells and humoral immunity (45–48). The semi-direct pathway allows linked help to be delivered by indirect pathway recognition of CD4 T cells to alloreactive CD8 T cells, which target the MHC-I alloantigen expressing cells within the graft after activation and thereby exhibit cytotoxic activity through expression and secretion of granzyme and perforin (36, 42, 49). Alloantigen recognition by Tregs with different pathways, however, regulates the hepatic immune “balance” substantially more favorable for “tolerance” (50). Therefore, interaction of alloreactive T cells and APCs will be the first and key step in regulating transplant outcome in LTx.
Crosstalk Between LSECs and Alloreactive T Cells
Within the liver allograft, there are many professional APCs such as DCs expressing low amounts of MHC antigens with co-stimulatory molecules and Kupffer cells (KCs) phagocytosing pathogens and secreting cytokines together with antigen processing and presenting (9, 51). Additionally, a large amount of non-professional APCs such as liver cells also interact with alloreactive T cells and contribute a lot to the liver transplant outcome. Composed of 50% of liver non-parenchymal cells, LSECs constitute a unique vascular bed with fenestrae organized in sieve plates without basal membrane in the liver. They interact directly with the immune cells and antigens in the blood flow, benefiting from the rich blood supply to the liver and the special liver sinusoid structure. Therefore, LSECs are also called “gatekeepers” of the hepatic immunity (52). Together with Kupffer cells, LSECs constitute the most powerful scavenger system in the body by the expression of pattern recognition receptors (PRRs) such as Toll-like receptors (TLR), scavenger receptors, and the potent endocytic capacity with their special fenestrae and loosely organized cell junctions (53, 54).
In addition to the potent endocytosis capacity, LSECs are also the unique liver-resident APCs by expressing both MHC-I and MHC-II molecules, which take up, process and present many antigens, including alloantigens to both CD8 and CD4 T cells within the liver graft. As shown in Figure 2, LSECs can take up alloantigens through PPRs, notably the mannose receptor (MR), process and transfer them to MHC-I for the priming of naïve CD8 T cells, which is called cross-presentation as MHC-I normally exhibit endogenous antigens rather than exogenous peptides (alloantigen as foreign antigen in transplant setting) (55, 56). However, the priming of naïve CD8 T cells by LSECs upregulates the expression of the co-inhibitory molecule B7-H1(PDL1) on LSECs whereas the expression of co-stimulatory molecule CD80/CD86 is not changed, thus the binding of B7-H1 on LSECs and PD-1 on naïve CD8 T cells leads to the apoptosis of the alloreactive CD8 T cells, creating therefore the tolerogenic environment within the liver graft. Interestingly, the LSECs-induced tolerance is highly correlated with antigen load and the strength of TCR stimulation in mice. Tolerance only occurs in low-dose antigen stimulation while high-dose antigen load results in the differentiation of effector memory T cell phenotype; this process is determined partly by IL-2 secretion of naïve CD8 T cells upon early antigen priming. Furthermore, exogenous IL-2 overrides B7-H1 mediated tolerance by LSECs and induces cytotoxic T lymphocytes (CTL) differentiation (57–59). Nevertheless, LSEC lectin (LSECtin), a member of the dendritic cell-specific ICAM-3 grabbing non-integrin (DC-SIGN) family, specifically recognizes activated T cells and negatively regulates the intrahepatic immune responses (60, 61).
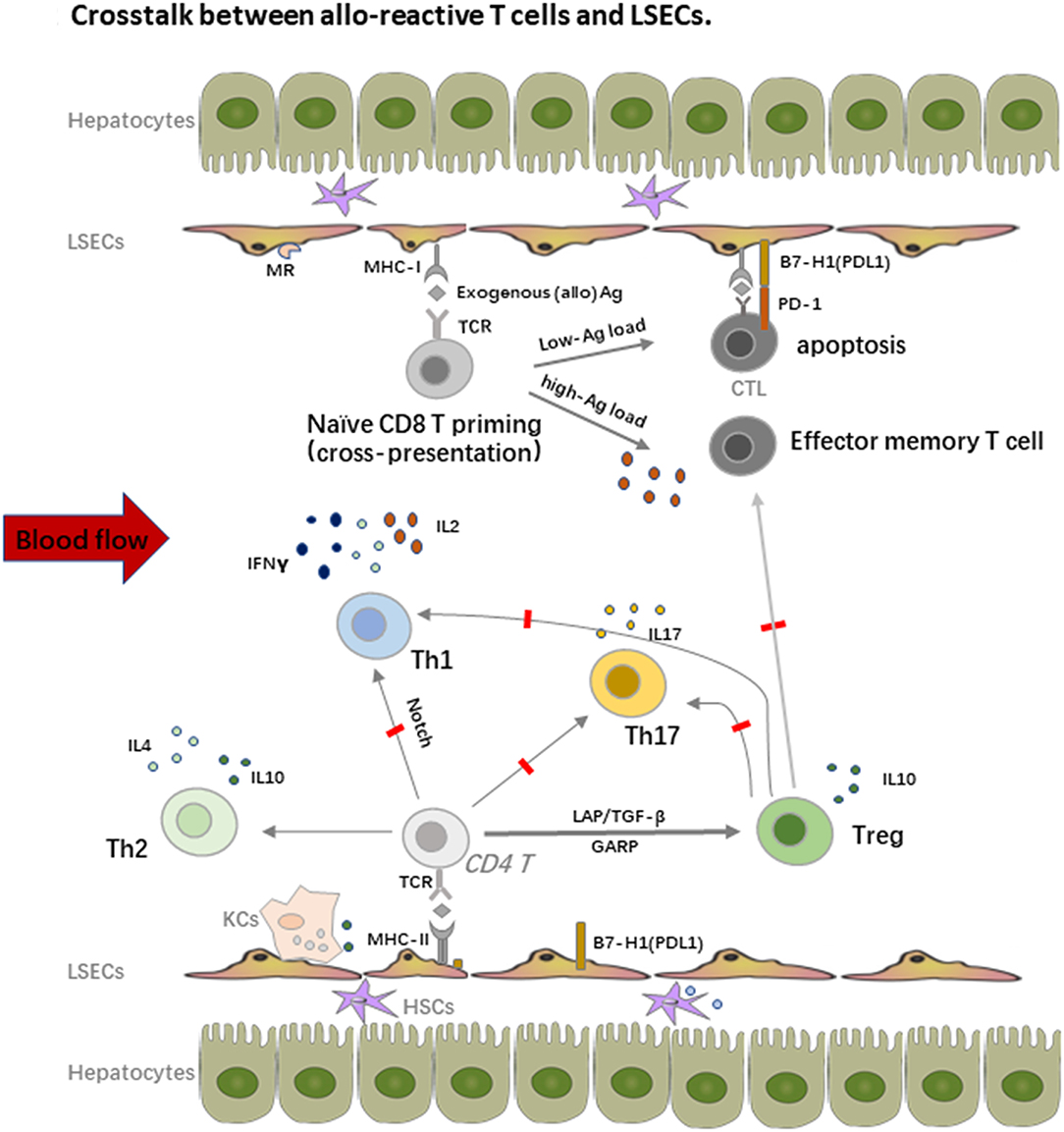
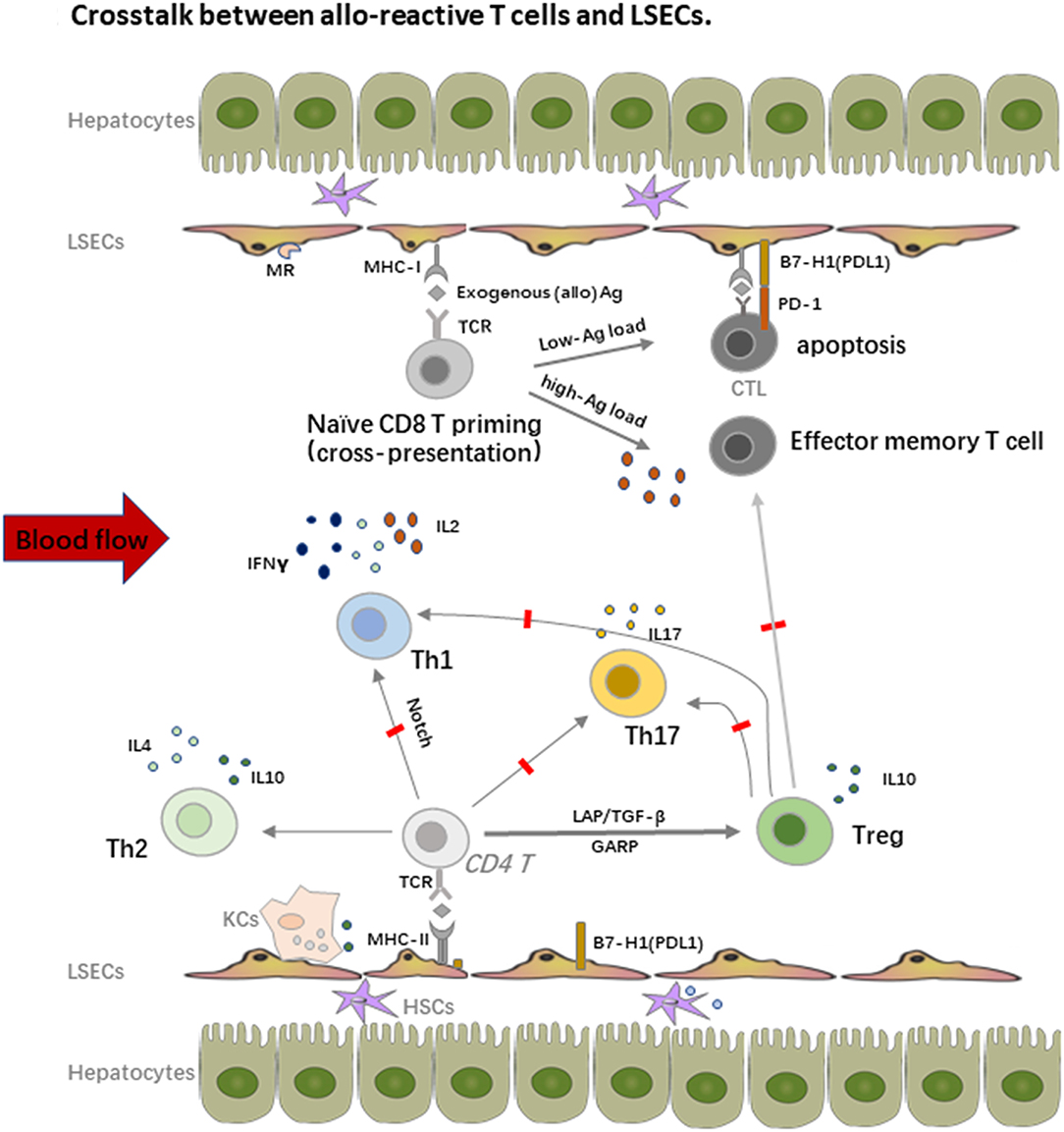
Figure 2. Crosstalk between alloreactive T cells and LSECs. LSECs constitute a unique vascular bed with fenestrae organized in sieve plates without basal membrane in the liver. They are the most powerful scavenger system by expression of pattern recognition receptors (PRRs), notably the mannose receptor (MR). On one hand, LSECs process and transfer the MHC-I to the naïve CD8 T cells, which is called “cross-dressing.” This priming process upregulates expression of the co-inhibitory molecule B7-H1(PDL1) on LSECs, whereas the expression of co-stimulatory molecule CD80/CD86 is not changed, thus the binding leads to the apoptosis of the alloreactive CD8 T cells. The LSECs induced tolerance is also highly correlated with antigen load and the strength of TCR stimulation. On the other hand, LSECs also prime naïve CD4 T cells with expression of MHC-II, especially under the inflammatory conditions, but fail to stimulate the proliferation of these cells due to the low expression of co-stimulatory molecules. LSECs also regulate the fate of naïve CD4 T cell differentiation within the liver graft. They suppress the differentiation of Th1 and Th17 cells but favor the enrichment of immune suppressive Th2 and Tregs, which promote the allograft tolerance.
Similar with CD8 T cells priming, LSECs can prime naïve CD4 T cells with expression of MHC-II, especially under inflammatory conditions, but fail to stimulate the proliferation of these cells due to the low expression of co-stimulatory molecules. Importantly, LSECs also regulate the fate of naïve CD4 T cell differentiation within the liver graft. Neumann et al. found that LSECs could suppress the differentiation of pro-inflammatory Th1 cells and promote the secretion of immune suppressive cytokines such as IL10 via the Notch pathway (62). As we addressed before, Tregs are another fundamental mediator for keeping allograft tolerance. There are several different Tregs including natural Tregs (nTregs), induced Tregs (iTregs), IL10 producing Type 1 regulatory T cells (Tr1 cells), and TGF-β producing Th3 cells. nTregs are mainly developed from the thymus while iTregs are induced from naïve T cells with the presence of a low amount of antigen and TGF-β. iTregs play an essential role in keeping immune homeostasis at mucosal interfaces with expression of probably a distinct TCR repertoire as nTregs (63). Under the condition of vast antigens in the liver and TGF-β secreted by DCs, hepatic iTregs are the major source of peripheral iTregs and lead to transplant tolerance together with nTregs in both humans and mice (17, 64–68). LSECs also promote cytokine secretion of the immune suppressive Th2 cells in addition to iTregs induction in animal models (69). Furthermore, in vitro stimulation of Th1 and Th17 by LSECs actively inhibits their capacity to secrete IFNγ and IL17, which is tightly correlated with the dominate inhibitory (B7-H1) over co-stimulatory (CD80/CD86) signals on LSECs and IL10 production by other tolerogenic cells such as DCs (70). As Th1 and Th17 cells are important mediators of transplant rejection post LTx (71, 72), the enrichment of Tregs contributes a lot to the tolerance induction as transient accumulation of total Tregs in peripheral blood of transplant recipients, especially non-rejection recipients at 1 or 2 weeks post LTx, was observed. Similar enrichment of Tregs was also proved in tolerogenic kidney transplant recipients, suggesting the priming of T cell response by the graft antigens (17, 67, 73).
Notably, the crosstalk between LSECs and T cells largely depends on cell-cell contact by different expression of adhesion molecules and chemokine receptors. Recruitment and accumulation of CD8 T cells within the liver depend primarily on TCR activated intercellular adhesion molecule 1 (ICAM1) expressed by LSECs and slightly on vascular cell adhesion molecule 1 (VCAM1), which does not need the recognition of intrahepatic antigens, thereby passively sequestering activated CD8 T cells (74). On the other hand, liver-resident T cells express lymphocyte function-associated antigen-1 (LFA-1) (CD11a or αLβ2 integrin) rather than CD103, an integrin that is required to retrain tissue-resident T cells in many epithelial tissues, to interact with ICAM1 on LSECs (75, 76). Chemokine receptor CXCL16 with its ligand CXCR6 is also involved in intrahepatic T cell and NKT cell recruitment, whereas Tregs bind to different chemokines due to their expression of CCR5 or CCR4; they are also reported to use distinct combination of adhesion receptors such as stabilin 1 to migrate cross LSECs (77).
Interactions of Hepatocytes and Alloreactive T Cells
Through interaction of immune cells with LSECs and adhesion cascade in the hepatic sinusoids, the survived lymphocytes from the LSECs immune surveillance can transmigrate across the LSECs line with help from the orchestra of chemokines and adhesion molecules through several different routes paracellularly, transcellularly, or intracellularly, to finally get a chance to crosstalk with hepatocytes (52). The paracrine factors that were secreted by hepatocytes also accelerate the recruitment of lymphocytes. The interaction of hepatocytes and immune cells plays an important role in inducing liver transplant tolerance. In general, hepatocytes mainly serve as non-professional APCs with expression of MHC-I to interact with CD8 T cells under physiological conditions while expression of MHC-II is also inducible under inflammatory conditions, especially in the presence of IFNγ. However, low expression of co-stimulatory molecules on hepatocytes leads to apoptosis of the alloreactive T cells (10). Paul-Heng et al. have found that direct recognition of hepatocyte expressed MHC-I alloantigen (cross presentation) is required for tolerance induction, whereas the indirect recognition of the processed and presented allogeneic peptide on MHC-II by CD4 T cells is not sufficient for tolerance induction although it can prolong the graft survival and generate Tregs to promote transplant tolerance (78, 79). Additionally, processing of the soluble antigens into peptide presented by MHC-I is impaired in hepatocytes lacking collectrin, which is an intracellular chaperone protein within the endoplasmic reticulum-Golgi intermediate compartment and positively regulated (80). Different from other liver cells, hepatocytes can produce exosomes to control the active T cells response and clear the activated T cells through the non-apoptotic way of suicidal emperipolesis (SE), which is a process leading to cell-in-cell structures and promotes cell death through degradation within endosomal/lysosomal compartments (Figure 3) (81, 82). Recently, Beringer et al. have found that the interaction of hepatoma HepaRG and human peripheral blood mononuclear cells (PBMCs) in the inflammatory response can be divided into two phases. At the early phase, PBMC-HepaRG interaction can modulate the T cell polarization into Th1 cells and suppress the differentiation into Th17 cells through direct cell-cell contact with increased secretion of IL6, IL8, CCL20, and MCP-1 (Figure 3), whereas the PBMC-hepatocyte crosstalk at the late phase may down-regulate the immune response with decreased expression of HLA-DR on hepatocytes to induce the immune tolerance in the liver (83). Moreover, it is not clear yet whether the similar kinetic interaction of alloreactive T cells and hepatocytes also exist in the LTx setting.
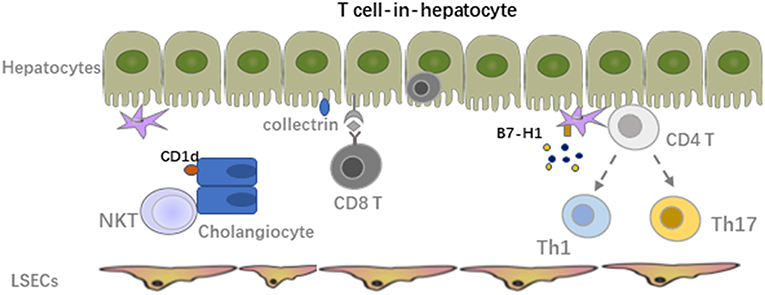
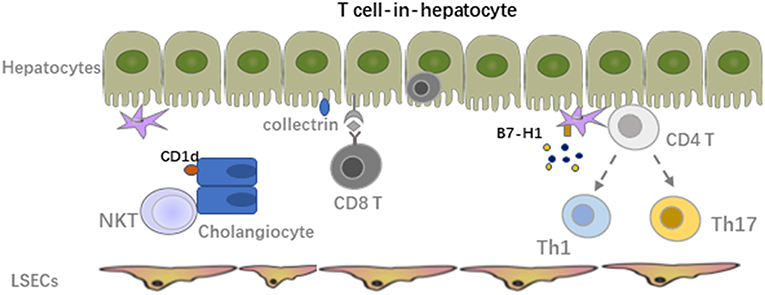
Figure 3. Crosstalk between alloreactive T cells and hepatocyte, HSCs, cholangiocytes. The survived lymphocytes from LSECs immune surveillance can transmigrate across the LSECs line to crosstalk with hepatocytes. The paracrine factors secreted by hepatocytes also accelerate the recruitment of lymphocytes. Hepatocytes mainly serve as non-professional APCs with expression of MHC-I to interact with CD8 T cells under physiological conditions while expression of MHC-II is also inducible under inflammatory condition especially in the presence of IFNγ. Interaction of HSCs with activated alloreactive T cells mainly lead to apoptosis of these T cells due to the low MHC-I expression. Expression of MHC-I like molecule CD1d on cholangiocytes can activate NKT cells and mediate inflammation in the bile ducts.
Interactions of HSCs, Cholangiocytes, and Immune Cells
Hepatic stellate cells (HSCs), also known as perisinusoidal cells or fat-storing cells, are crucial in liver inflammation and fibrosis by producing inflammatory and fibrotic mediators. In the context of LTx, migrating host immune cells also interact with graft liver resident cells. Both cell-cell contact and soluble cytokines or factors contribute to the graft function and transplant outcome. Inflammatory cell derived IL17A induced HSC to express collagen I directly and TGF-β from activated KCs induced expression of collagen I on HSCs indirectly, promoting the graft fibrosis progression (84). Activated HSCs produce inflammatory cytokines and chemotactic factors to accelerate the migration and deposition of immune cells, which could be further enhanced by paracrine signals from damaged hepatocytes (85–87). However, due to the low amount of MHC-I expression and co-inhibitory molecule B7-H1 on HSCs, interaction of HSCs with activated alloreactive T cells mainly leads to apoptosis of these T cell. In addition, mature HSCs can stimulate allogeneic Treg proliferation with the manner of cell-cell contact and enhance the suppressive capacity of Tregs regarding inhibiting of Teff proliferation in vitro. Adoptive transfer of HSC-stimulated Tregs significantly reduced liver injury in mice with autoimmune hepatitis by modulating the balance between Tregs and Th17 cell responses (88).
Cholangiocytes express MHC-I under physiological conditions and a low amount of MHC-II only in the context of inflammation (89). It was reported that through expression of MHC-I like molecule CD1d, murine cholangiocytes could present both exogenous (cross-presentation) and endogenous lipid antigens to NKT cells and activate them to mediate inflammation in the bile ducts. The human cholangiocytes also present exogenous antigens in a CD1d-restricted way to invariant NKT cells. However, CD1d expression was down-regulated in the biliary epithelium of patients with late primary sclerosing cholangitis and primary biliary cirrhosis compared to healthy controls, suggesting their potential role in the pathology of these diseases (90, 91). On biliary epithelial cells (BECs) in biliary atresia patients, increased ICAM-1 expression was also observed in association with MHC-I, but not MHC-II. The major lymphocytes within the portal tracts are CD4 T cells expressing LFA-1, indicating the potential crosstalk between them (92). MHC-I expression level on cholangiocytes might correlate with cholangitis post LTx. Interestingly, BECs express a relatively higher amount of MHC-I compared with other liver cells (12).
Therapeutic Targets for Liver Transplant Tolerance Induction
Operational tolerance, characterized with stable graft function in the absence of IS for at least 1 year, is the final goal of all allogenic solid organ transplantation (SOT). To achieve this, several approaches for immune modulation, including adoptive cell therapy, have been conducted in the clinical trials. We and others have showed that both recipient and donor Tregs play an essential role in maintaining the graft tolerance in SOT (17, 18, 93–95). Adoptive Treg-based therapy is a very promising approach to support allograft acceptance with minimizing or potentially eliminating IS treatment. A phase II international multicenter proof-of-concept clinical trial of Treg therapy for SOT patients has been conducted in the European Union (The ONE Study). Our group have shown that nTregs from even end-stage renal disease patients could be expanded ex-vivo for adoptive cell therapy, whereas alloantigen specific Tregs exhibit superior immune suppressive capacity for tolerance induction (96, 97). Moreover, adoptive Treg transfer in the inflammatory phase of viral-induced myocarditis protects the heart against inflammatory damage and fibrosis via modulation of monocyte differentiation in favor of the anti-inflammatory Ly6ClowCCR2lowCx3Cr1high subset (98). In the liver transplant setting, Todo et al. have published a very exciting pilot study that 7 of the 10 liver transplant recipients receiving a single dose of donor antigen specific Tregs and splenectomy become operationally tolerant (99). Several clinical trials for adoptive cell therapy employing either ex vivo expanded polyclonal Tregs or alloantigen specific Tregs are also being conducted worldwide. The ThRIL trial at King’s College Hospital, UK [clinical trials.gov NCT02166177] utilizes polyclonal Tregs in their therapeutic setting. The DeLTA and ARTEMIS trials at University of California, San Francisco, USA, use donor antigen reactive Tregs for tolerance induction in both deceased donor LTx [NCT02188719] and living donor LTx [NCT02474199]. These clinical trials will not only show the efficiency and safety of Treg therapy but also indicate the survival and homing of these adoptively transferred cells as they are labeled with deuterium (100). Another clinical trial at Nanjing Medical University, China, utilizes donor antigen specific Tregs for chronic rejections in LTx patients at early and late time points, with multiple Treg injections and IS withdrawal [NCT01624077] (101).
In addition to ex vivo expansion of Tregs for adoptive cell therapy, other strategies regarding in vivo expansion of Tregs are also very appealing. For instance, low-dose IL-2 administration could expand Tregs in vivo up to 8 times without a significant increase in Teff cells because Tregs express a higher amount of IL-2 receptor α-chain (CD25) and thus respond to a very low amount of IL-2 while Teff could not. This brings the possibility to expand Tregs pool in vivo without requirement of very expensive and large-scale GMP facilities for clinical grade Treg products (102). Low dose IL-2 also restores Treg homeostasis or dysfunction in chronic GvHD patients (103, 104). A corresponding phase IV clinical trial, LITE Trial (NCT02949492), is in progress at King’s College London. Scientists there are using low dose IL-2 to promote the selective expansion of endogenous Tregs in liver transplant recipients at the time of immunosuppression (101). Recently, Ratnasothy et al. even showed that IL-2 treatment in mice preferentially enhances the proliferation of the adoptively transferred allospecific Tregs in an antigen-dependent manner and increases the expression of regulatory-related markers, such as CTLA4 and inducible co-stimulator (ICOS). Based on this, combination therapy of both low-dose IL-2 and adoptively transferred alloantigen specific Tregs could provide an appropriate condition to enhance the immunoregulation toward alloimmune response in clinical transplantation (105). Low-dose IL-2 enriched Treg therapy is also investigated intensively in autoimmune diseases and GvHD after hematopoietic stem cell transplantation (106–109).
Notably, as antigen specific Tregs are superior to polyclonal Tregs in controlling Teff responses, improving approximately 100-fold of the efficacy, and theoretically safer due to avoiding bystander compromised immunity (110, 111), it is more appealing to use this Treg population for adoptive Tregs therapy. However, expansion of these Tregs in vitro is a big obstacle for clinical translation. Therefore, engineering human T cells to express a chimeric antigen receptor (CAR) is a new approach to create antigen specific T cells. For instance, autoantigen-based chimeric immunoreceptors can direct T cells to kill autoreactive B lymphocytes through the specificity of the B cell receptor (BCR) (112). Meanwhile, CAR Tregs can also be generated with CAR technology to develop alloantigen specific Tregs, which have showed potent and markedly enhanced therapeutic potential for the protection of allografts (113–115). Co-administration of antigen with tolerogenic nanoparticles (tNPs), which comprised of biodegradable polymers with encapsulated rapamycin, could inhibit ag-specific transgenic Teff proliferation and induce ag-specific Tregs. This suggests another potential strategy to expand ag-specific Tregs in vivo and suppress T cell-mediated autoimmunity or graft rejection (116–118). On the contrary, Treg plasticity refers to their capacity to produce inflammatory cytokines and lose FoxP3 expression (119, 120). In this case, they could transform into pathogenic Teff cells, thus contributing to disease pathogenesis, which might represent a risk for adoptive Tregs therapy. Based on these concerns, genetic “editing” through CRISPR-associated protein 9 (Cas9) system could generate optimal Tregs while ensuring stability (121, 122). However, our group have recently published that gene-editing with CRISPR-Cas9 system might cause significant safety issues because of the pre-existing ubiquitous effector T cell response directed toward the Streptococcus pyogenes (SpCas9) within healthy humans. Therefore, modification of Tregs with the CRISPR-Cas9 system still needs further careful evaluation (123).
Similar to Tregs, regulatory B cells (Bregs) function as a form of active immune regulation, which was first reported experimentally through anti-CD45RB treatment of mice receiving a cardiac allograft (124). Moreover, the field of Breg-mediated tolerance is relatively immature and their function is somehow also related with Tregs (125, 126). Regulatory DCs (DCregs) with capacity to suppress allograft rejection and promote transplant tolerance in pre-clinical models can readily be generated from bone marrow precursors or circulating blood monocytes. Donor-derived DCregs are short-lived but can induce robust donor-specific T cell hyporesponsiveness. Infusion of donor-derived DCregs could achieve IS withdrawal in patients 18 months post LTx (38, 127). Furthermore, down-regulation of HLA-1 expression level on hepatocytes can reduce the strength of allogeneic immune responses and improve the graft survival. Alternatively, gene transfer of alloantigen to hepatocytes induces the expansion of CD8 Tregs, which further prevent the allograft rejection in mice pancreatic islets transplantation. These gene-modified hepatocytes may also provide some possible tolerance induction strategy in the future (128, 129).
Summary and Outlook
Based on the alloimmune responses mediated transplant rejection, interactions of alloreactive T cells with both innate immune cells and liver cells including hepatocytes, LSECs, HSCs, and cholangiocytes contribute dramatically to the transplant outcome. The capacity of alloantigen presenting and inflammatory mediator secretion by liver cells dominates the fate of alloreactive T cell differentiation and transplant outcome. As Tregs play an essential role in inducing and maintaining the allograft tolerance, Treg based therapy either with adoptively transferred ex vivo expanded Tregs or low-dose IL-2 in vivo enriched Tregs pool is very promising and appealing for clinical translation. However, more efficient Treg expansion protocols have to be developed and evaluated to improve the efficiency of the therapy and reduce the cost for the clinical cell products. In addition, combination of several tolerance induction strategies might provide synergistic results, but more clinical studies from multiple centers still need to be conducted for successful translation from bench to bedside.
Author Contributions
All authors listed have made a substantial, direct and intellectual contribution to the work, and approved it for publication.
Funding
This work was supported by China Postdoctoral Science Foundation Grant (No. 2019M653663) and grants from the National Nature Science Foundation of China (Nos. 81770491 and 81870445) and the Ministry of Education Innovation Team Development Program of China (No. IRT16R57).
Conflict of Interest
The authors declare that the research was conducted in the absence of any commercial or financial relationships that could be construed as a potential conflict of interest.
References
2. Londono MC, Rimola A, O’Grady J, Sanchez-Fueyo A. Immunosuppression minimization vs. complete drug withdrawal in liver transplantation. J Hepatol. (2013) 59:872–9. doi: 10.1016/j.jhep.2013.04.003
3. Morris H, DeWolf S, Robins H, Sprangers B, LoCascio SA, Shonts BA, et al. Tracking donor-reactive T cells: evidence for clonal deletion in tolerant kidney transplant patients. Sci Transl Med. (2015) 7:272ra210. doi: 10.1126/scitranslmed.3010760
4. Zimmerer JM, Horne PH, Fisher MG, Pham TA, Lunsford KE, Ringwald BA, et al. Unique CD8+ T cell-mediated immune responses primed in the liver. Transplantation. (2016) 100:1907–15. doi: 10.1097/TP.0000000000001290
7. Freitas-Lopes MA, Mafra K, David BA, Carvalho-Gontijo R, Menezes GB. Differential location and distribution of hepatic immune cells. Cells. (2017) 6:E48. doi: 10.3390/cells6040048
10. Mehrfeld C, Zenner S, Kornek M, Lukacs-Kornek V. The contribution of non-professional antigen-presenting cells to immunity and tolerance in the liver. Front Immunol. (2018) 9:635. doi: 10.3389/fimmu.2018.00635
11. Musat AI, Agni RM, Wai PY, Pirsch JD, Lorentzen DF, Powell A, et al. The significance of donor-specific HLA antibodies in rejection and ductopenia development in ABO compatible liver transplantation. Am J Transplant. (2011) 11:500–10. doi: 10.1111/j.1600-6143.2010.03414.x
12. Demetris AJ, Bellamy CO, Gandhi CR, Prost S, Nakanuma Y, Stolz DB. Functional immune anatomy of the liver-as an allograft. Am J Transplant. (2016) 16:1653–80. doi: 10.1111/ajt.13749
13. Hogen R, DiNorcia J, Dhanireddy K. Antibody-mediated rejection: what is the clinical relevance? Curr Opin Organ Transplant. (2017) 22:97–104. doi: 10.1097/MOT.0000000000000391
14. Kim H, Kim H, Lee SK, Jin XL, Kim TJ, Park C, et al. Memory T cells are significantly increased in rejected liver allografts of rhesus monkeys. Liver Transpl. (2018) 24:256–68. doi: 10.1002/lt.24983
15. Jimbo K, Konuma T, Watanabe E, Kohara C, Mizukami M, Nagai E, et al. T memory stem cells after allogeneic haematopoietic cell transplantation: unique long-term kinetics and influence of chronic graft-versus-host disease. Br J Haematol. (2019) 186:866–78. doi: 10.1111/bjh.15995
16. Soares MV, Azevedo RI, Ferreira IA, Bucar S, Ribeiro AC, Vieira A, et al. Naive and stem cell memory T cell subset recovery reveals opposing reconstitution patterns in CD4 and CD8 T cells in chronic graft vs. host disease. Front Immunol. (2019) 10:334. doi: 10.3389/fimmu.2019.00334
17. Braza F, Dugast E, Panov I, Paul C, Vogt K, Pallier A, et al. Central role of CD45RA- Foxp3hi memory regulatory T cells in clinical kidney transplantation tolerance. J Am Soc Nephrol. (2015) 26:1795–805. doi: 10.1681/ASN.2014050480
18. Lei H, Kuchenbecker L, Streitz M, Sawitzki B, Vogt K, Landwehr-Kenzel S, et al. Human CD45RA(-) FoxP3(hi) memory-type regulatory T cells show distinct TCR repertoires with conventional T cells and play an important role in controlling early immune activation. Am J Transplant. (2015) 15:2625–35. doi: 10.1111/ajt.13315
20. Arstila TP, Casrouge A, Baron V, Even J, Kanellopoulos J, Kourilsky P. A direct estimate of the human alphabeta T cell receptor diversity. Science. (1999) 286:958–61. doi: 10.1126/science.286.5441.958
21. Elkins WL, Guttmann RD. Pathogenesis of a local graft versus host reaction: immunogenicity of circulating host leukocytes. Science. (1968) 159:1250–1. doi: 10.1126/science.159.3820.1250
22. Talmage DW, Dart G, Radovich J, Lafferty KJ. Activation of transplant immunity: effect of donor leukocytes on thyroid allograft rejection. Science. (1976) 191:385–8. doi: 10.1126/science.1082167
23. Lakkis FG, Arakelov A, Konieczny BT, Inoue Y. Immunologic ‘ignorance’ of vascularized organ transplants in the absence of secondary lymphoid tissue. Nat Med. (2000) 6:686–8. doi: 10.1038/76267
24. Schlitt HJ, Raddatz G, Steinhoff G, Wonigeit K, Pichlmayr R. Passenger lymphocytes in human liver allografts and their potential role after transplantation. Transplantation. (1993) 56:951–5. doi: 10.1097/00007890-199310000-00033
25. Sun J, McCaughan GW, Gallagher ND, Sheil AG, Bishop GA. Deletion of spontaneous rat liver allograft acceptance by donor irradiation. Transplantation. (1995) 60:233–6. doi: 10.1097/00007890-199508000-00004
26. Zhang Y, Zhao H, Bo L, Yang Y, Lu X, Sun J, et al. Total body irradiation of donors can alter the course of tolerance and induce acute rejection in a spontaneous tolerance rat liver transplantation model. Sci China Life Sci. (2012) 55:774–81. doi: 10.1007/s11427-012-4370-3
28. Starzl TE, Demetris AJ, Trucco M, Ramos H, Zeevi A, Rudert WA, et al. Systemic chimerism in human female recipients of male livers. Lancet. (1992) 340:876–7. doi: 10.1016/0140-6736(92)93286-V
29. Starzl TE, Demetris AJ, Trucco M, Murase N, Ricordi C, Ildstad S, et al. Cell migration and chimerism after whole-organ transplantation: the basis of graft acceptance. Hepatology. (1993) 17:1127–52. doi: 10.1002/hep.1840170629
30. Ali JM, Negus MC, Conlon TM, Harper IG, Qureshi MS, Motallebzadeh R, et al. Diversity of the CD4 T cell alloresponse: the short and the long of it. Cell Rep. (2016) 14:1232–45. doi: 10.1016/j.celrep.2015.12.099
31. Valujskikh A, Zhang Q, Heeger PS. CD8 T cells specific for a donor-derived, self-restricted transplant antigen are nonpathogenic bystanders after vascularized heart transplantation in mice. J Immunol. (2006) 176:2190–6. doi: 10.4049/jimmunol.176.4.2190
33. Ono Y, Perez-Gutierrez A, Nakao T, Dai H, Camirand G, Yoshida O. et al. Graft-infiltrating PD-L1hi cross-dressed dendritic cells regulate antidonor T cell responses in mouse liver transplant tolerance. Hepatology. (2018) 67:1499–515. doi: 10.1002/hep.29529
34. Brown K, Sacks SH, Wong W. Coexpression of donor peptide/recipient MHC complex and intact donor MHC: evidence for a link between the direct and indirect pathways. Am J Transplant. (2011) 11:826–31. doi: 10.1111/j.1600-6143.2011.03437.x
35. Sivaganesh S, Harper SJ, Conlon TM, Callaghan CJ, Saeb-Parsy K, Negus MC, et al. Copresentation of intact and processed MHC alloantigen by recipient dendritic cells enables delivery of linked help to alloreactive CD8 T cells by indirect-pathway CD4 T cells. J Immunol. (2013) 190:5829–38. doi: 10.4049/jimmunol.1300458
36. Harper SJ, Ali JM, Wlodek E, Negus MC, Harper IG, Chhabra M, et al. CD8 T-cell recognition of acquired alloantigen promotes acute allograft rejection. Proc Natl Acad Sci USA. (2015) 112:12788–93. doi: 10.1073/pnas.1513533112
37. Smyth LA, Lechler RI, Lombardi G. Continuous acquisition of MHC:peptide complexes by recipient cells contributes to the generation of anti-graft CD8(+) T cell immunity. Am J Transplant. (2017) 17:60–8. doi: 10.1111/ajt.13996
38. Thomson AW, Humar A, Lakkis FG, Metes DM. Regulatory dendritic cells for promotion of liver transplant operational tolerance: rationale for a clinical trial and accompanying mechanistic studies. Hum Immunol. (2018) 79:314–21. doi: 10.1016/j.humimm.2017.10.017
39. Russo V, Zhou D, Sartirana C, Rovere P, Villa A, Rossini S, et al. Acquisition of intact allogeneic human leukocyte antigen molecules by human dendritic cells. Blood. (2000) 95:3473–7. doi: 10.1182/blood.V95.11.3473
40. Harshyne LA, Watkins SC, Gambotto A, Barratt-Boyes SM. Dendritic cells acquire antigens from live cells for cross-presentation to CTL. J Immunol. (2001) 166:3717–23. doi: 10.4049/jimmunol.166.6.3717
41. Game DS, Rogers NJ, Lechler RI. Acquisition of HLA-DR and costimulatory molecules by T cells from allogeneic antigen presenting cells. Am J Transplant. (2005) 5:1614–25. doi: 10.1111/j.1600-6143.2005.00916.x
42. Liu Q, Rojas-Canales DM, Divito SJ, Shufesky WJ, Stolz DB, Erdos G, et al. Donor dendritic cell-derived exosomes promote allograft-targeting immune response. J Clin Invest. (2016) 126:2805–20. doi: 10.1172/JCI84577
43. Marino J, Babiker-Mohamed MH, Crosby-Bertorini P, Paster JT, LeGuern C, Germana S, et al. Donor exosomes rather than passenger leukocytes initiate alloreactive T cell responses after transplantation. Sci Immunol. (2016) 1:aaf8759. doi: 10.1126/sciimmunol.aaf8759
44. Morelli AE, Bracamonte-Baran W, Burlingham WJ. Donor-derived exosomes: the trick behind the semidirect pathway of allorecognition. Curr Opin Organ Transplant. (2017) 22:46–54. doi: 10.1097/MOT.0000000000000372
45. Conlon TM, Saeb-Parsy K, Cole JL, Motallebzadeh R, Qureshi MS, Rehakova S, et al. Germinal center alloantibody responses are mediated exclusively by indirect-pathway CD4 T follicular helper cells. J Immunol. (2012) 188:2643–52. doi: 10.4049/jimmunol.1102830
46. Ali J, Bolton E, Saeb-Parsy K, Bradley JA, Pettigrew G. Targeting indirect pathway CD4 T-cell alloresponses in the prevention of chronic transplant rejection. Lancet. (2015) 385 (Suppl. 1):S17. doi: 10.1016/S0140-6736(15)60332-4
48. Alsughayyir J, Chhabra M, Qureshi MS, Mallik M, Ali JM, Gamper I, et al. Relative frequencies of alloantigen-specific helper CD4 T cells and B cells determine mode of antibody-mediated allograft rejection. Front Immunol. (2018) 9:3039. doi: 10.3389/fimmu.2018.03039
49. Obst R, van Santen HM, Mathis D, Benoist C. Antigen persistence is required throughout the expansion phase of a CD4(+) T cell response. J Exp Med. (2005) 201:1555–65. doi: 10.1084/jem.20042521
50. Vaikunthanathan T, Safinia N, Lombardi G. Optimizing regulatory T cells for therapeutic application in human organ transplantation. Curr Opin Organ Transplant. (2018) 23:516–23. doi: 10.1097/MOT.0000000000000561
51. He W, Chen L, Zheng L, Luo L, Gao L. Prolonged survival effects induced by immature dendritic cells and regulatory T cells in a rat liver transplantation model. Mol Immunol. (2016) 79:92–7. doi: 10.1016/j.molimm.2016.10.004
52. Shetty S, Lalor PF, Adams DH. Liver sinusoidal endothelial cells – gatekeepers of hepatic immunity. Nat Rev Gastroenterol Hepatol. (2018) 15:555–67. doi: 10.1038/s41575-018-0020-y
55. Limmer A, Ohl J, Kurts C, Ljunggren HG, Reiss Y, Groettrup M, et al. Efficient presentation of exogenous antigen by liver endothelial cells to CD8+ T cells results in antigen-specific T-cell tolerance. Nat Med. (2000) 6:1348–54. doi: 10.1038/82161
56. Burgdorf S, Kautz A, Bohnert V, Knolle PA, Kurts C. Distinct pathways of antigen uptake and intracellular routing in CD4 and CD8 T cell activation. Science. (2007) 316:612–6. doi: 10.1126/science.1137971
57. Schurich A, Berg M, Stabenow D, Bottcher J, Kern M, Schild HJ, et al. Dynamic regulation of CD8 T cell tolerance induction by liver sinusoidal endothelial cells. J Immunol. (2010) 184:4107–14. doi: 10.4049/jimmunol.0902580
58. Robinson KA, Orent W, Madsen JC, Benichou G. Maintaining T cell tolerance of alloantigens: lessons from animal studies. Am J Transplant. (2018) 18:1843–56. doi: 10.1111/ajt.14984
59. Tolksdorf F, Mikulec J, Geers B, Endig J, Sprezyna P, Heukamp LC, et al. The PDL1-inducible GTPase Arl4d controls T effector function by limiting IL-2 production. Sci Rep. (2018) 8:16123. doi: 10.1038/s41598-018-34522-4
60. Tang L, Yang J, Liu W, Tang X, Chen J, Zhao D, et al. Liver sinusoidal endothelial cell lectin, LSECtin, negatively regulates hepatic T-cell immune response. Gastroenterology. (2009) 137:1498–508.e1-5. doi: 10.1053/j.gastro.2009.07.051
61. Yang Z, Li Q, Wang X, Jiang X, Zhao D, Lin X, et al. C-type lectin receptor LSECtin-mediated apoptotic cell clearance by macrophages directs intestinal repair in experimental colitis. Proc Natl Acad Sci USA. (2018) 115:11054–9. doi: 10.1073/pnas.1804094115
62. Neumann K, Rudolph C, Neumann C, Janke M, Amsen D, Scheffold A. Liver sinusoidal endothelial cells induce immunosuppressive IL-10-producing Th1 cells via the Notch pathway. Eur J Immunol. (2015) 45:2008–16. doi: 10.1002/eji.201445346
63. Schwele S, Fischer AM, Brestrich G, Wlodarski MW, Wagner L, Schmueck M, et al. Cytomegalovirus-specific regulatory and effector T cells share TCR clonality–possible relation to repetitive CMV infections. Am J Transplant. (2012) 12:669–81. doi: 10.1111/j.1600-6143.2011.03842.x
64. Carambia A, Freund B, Schwinge D, Heine M, Laschtowitz A, Huber S, et al. TGF-beta-dependent induction of CD4(+)CD25(+)Foxp3(+) Tregs by liver sinusoidal endothelial cells. J Hepatol. (2014) 61:594–9. doi: 10.1016/j.jhep.2014.04.027
65. Taubert R, Danger R, Londono MC, Christakoudi S, Martinez-Picola M, Rimola A, et al. Hepatic infiltrates in operational tolerant patients after liver transplantation show enrichment of regulatory T cells before proinflammatory genes are downregulated. Am J Transplant. (2016) 16:1285–93. doi: 10.1111/ajt.13617
67. Revilla-Nuin B, de Bejar A, Martinez-Alarcon L, Herrero JI, Martinez-Caceres CM, Ramirez P, et al. Differential profile of activated regulatory T cell subsets and microRNAs in tolerant liver transplant recipients. Liver Transpl. (2017) 23:933–45. doi: 10.1002/lt.24691
68. Wu H, Xu X, Li J, Gong J, Li M. TIM4 blockade of KCs combined with exogenous TGFbeta injection helps to reverse acute rejection and prolong the survival rate of mice receiving liver allografts. Int J Mol Med. (2018) 42:346–58. doi: 10.3892/ijmm.2018.3606
69. Liu Q, Wang X, Liu X, Kumar S, Gochman G, Ji Y, et al. Use of polymeric nanoparticle platform targeting the liver to induce tregs-mediated antigen-specific immune tolerance in a pulmonary allergen sensitization model. ACS Nano. (2019) 13:4778–94. doi: 10.1021/acsnano.9b01444
70. Carambia A, Frenzel C, Bruns OT, Schwinge D, Reimer R, Hohenberg H, et al. Inhibition of inflammatory CD4 T cell activity by murine liver sinusoidal endothelial cells. J Hepatol. (2013) 58:112–8. doi: 10.1016/j.jhep.2012.09.008
71. Shi G, Zeng Q, Zhao Z, Li W, Dou J. Variation of CD4(+)CD25(+)Foxp3(+) regulatory T cells and Th17 cells in the peripheral blood of human liver allograft patients with long-term survival. Transplant Proc. (2017) 49:1834–40. doi: 10.1016/j.transproceed.2017.06.026
72. Wang K, Song ZL, Wu B, Zhou CL, Liu W, and Gao W. The T-helper cells 17 instead of Tregs play the key role in acute rejection after pediatric liver transplantation. Pediatr Transplant. (2019) 23:e13363. doi: 10.1111/petr.13363
73. Haarer J, Riquelme P, Hoffmann P, Schnitzbauer A, Schlitt HJ, Sawitzki B, et al. Early enrichment and restitution of the peripheral blood tregs pool is associated with rejection-free stable immunosuppression after liver transplantation. Transplantation. (2016) 100:e39–40. doi: 10.1097/TP.0000000000001190
75. Mackay LK, Rahimpour A, Ma JZ, Collins N, Stock AT, Hafon ML, et al. The developmental pathway for CD103(+)CD8+ tissue-resident memory T cells of skin. Nat Immunol. (2013) 14:1294–301. doi: 10.1038/ni.2744
76. McNamara HA, Cai Y, Wagle MV, Sontani Y, Roots CM, Miosge LA, et al. Up-regulation of LFA-1 allows liver-resident memory T cells to patrol and remain in the hepatic sinusoids. Sci Immunol. (2017) 2:eaaj1996. doi: 10.1126/sciimmunol.aaj1996
78. Warren A, Le Couteur DG, Fraser R, Bowen DG, McCaughan GW, Bertolino P. T lymphocytes interact with hepatocytes through fenestrations in murine liver sinusoidal endothelial cells. Hepatology. (2006) 44:1182–90. doi: 10.1002/hep.21378
79. Paul-Heng M, Leong M, Cunningham E, Bunker DLJ, Bremner K, Wang Z, et al. Direct recognition of hepatocyte-expressed MHC class I alloantigens is required for tolerance induction. JCI Insight. (2018) 3:97500. doi: 10.1172/jci.insight.97500
80. Dolina JS, Cechova S, Rudy CK, Sung SJ, Tang WW, Lee J, et al. Cross-presentation of soluble and cell-associated antigen by murine hepatocytes is enhanced by collectrin expression. J Immunol. (2017) 198:2341–51. doi: 10.4049/jimmunol.1502234
81. Sierro F, Tay SS, Warren A, Le Couteur DG, McCaughan GW, Bowen DG, et al. Suicidal emperipolesis: a process leading to cell-in-cell structures, T cell clearance and immune homeostasis. Curr Mol Med. (2015) 15:819–27. doi: 10.2174/1566524015666151026102143
82. Holman NS, Church RJ, Nautiyal M, Rose KA, Thacker SE, Otieno MA, et al. Hepatocyte-derived exosomes promote liver immune tolerance: possible implications for idiosyncratic drug-induced liver injury. Toxicol Sci. (2019) 170:499–508. doi: 10.1093/toxsci/kfz112
83. Beringer A, Molle J, Bartosch B, Miossec P. Two phase kinetics of the inflammatory response from hepatocyte-peripheral blood mononuclear cell interactions. Sci Rep. (2019) 9:8378. doi: 10.1038/s41598-019-44840-w
84. Meng F, Wang K, Aoyama T, Grivennikov SI, Paik Y, Scholten D, et al. Interleukin-17 signaling in inflammatory, Kupffer cells, and hepatic stellate cells exacerbates liver fibrosis in mice. Gastroenterology. (2012) 143:765–76.e763. doi: 10.1053/j.gastro.2012.05.049
85. Marra F, Valente AJ, Pinzani M, Abboud HE. Cultured human liver fat-storing cells produce monocyte chemotactic protein-1. Regulation by proinflammatory cytokines. J Clin Invest. (1993) 92:1674–80. doi: 10.1172/JCI116753
86. Thirunavukkarasu C, Watkins SC, Gandhi CR. Mechanisms of endotoxin-induced NO, IL-6, and TNF-alpha production in activated rat hepatic stellate cells: role of p38 MAPK. Hepatology. (2006) 44:389–98. doi: 10.1002/hep.21254
87. Beringer A, Miossec P. IL-17 and TNF-alpha co-operation contributes to the proinflammatory response of hepatic stellate cells. Clin Exp Immunol. (2019) 198:111–20. doi: 10.1111/cei.13316
88. Huang H, Deng Z. Adoptive transfer of regulatory T cells stimulated by allogeneic hepatic stellate cells mitigates liver injury in mice with concanavalin A-induced autoimmune hepatitis. Biochem Biophys Res Commun. (2019) 512:14–21. doi: 10.1016/j.bbrc.2019.02.147
89. Feng J, Li M, Gu W, Tang H, Yu S. The aberrant expression of HLA-DR in intrahepatic bile ducts in patients with biliary atresia: an immunohistochemistry and immune electron microscopy study. J Pediatr Surg. (2004) 39:1658–62. doi: 10.1016/j.jpedsurg.2004.07.010
90. Schrumpf E, Tan C, Karlsen TH, Sponheim J, Bjorkstrom NK, Sundnes O, et al. The biliary epithelium presents antigens to and activates natural killer T cells. Hepatology. (2015) 62:1249–59. doi: 10.1002/hep.27840
91. Berntsen NL, Fosby B, Tan C, Reims HM, Ogaard J, Jiang X, et al. Natural killer T cells mediate inflammation in the bile ducts. Mucosal Immunol. (2018) 11:1582–90. doi: 10.1038/s41385-018-0066-8
92. Dillon PW, Belchis D, Minnick K, Tracy T. Differential expression of the major histocompatibility antigens and ICAM-1 on bile duct epithelial cells in biliary atresia. Tohoku J Exp Med. (1997) 181:33–40. doi: 10.1620/tjem.181.33
93. Martinez-Llordella M, Puig-Pey I, Orlando G, Ramoni M, Tisone G, Rimola A, et al. Multiparameter immune profiling of operational tolerance in liver transplantation. Am J Transplant. (2007) 7:309–19. doi: 10.1111/j.1600-6143.2006.01621.x
94. Pons JA, Revilla-Nuin B, Baroja-Mazo A, Ramirez P, Martinez-Alarcon L, Sanchez-Bueno F, et al. FoxP3 in peripheral blood is associated with operational tolerance in liver transplant patients during immunosuppression withdrawal. Transplantation. (2008) 86:1370–8. doi: 10.1097/TP.0b013e318188d3e6
95. Savage TM, Shonts BA, Obradovic A, Dewolf S, Lau S, Zuber J, et al. Early expansion of donor-specific Tregs in tolerant kidney transplant recipients. JCI Insight. (2018) 3:124086. doi: 10.1172/jci.insight.124086
96. Landwehr-Kenzel S, Issa F, Luu SH, Schmuck M, Lei H, Zobel A, et al. Novel GMP-compatible protocol employing an allogeneic B cell bank for clonal expansion of allospecific natural regulatory T cells. Am J Transplant. (2014) 14:594–606. doi: 10.1111/ajt.12629
97. Landwehr-Kenzel S, Zobel A, Hoffmann H, Landwehr N, Schmueck-Henneresse M, Schachtner T, et al. Ex vivo expanded natural regulatory T cells from patients with end-stage renal disease or kidney transplantation are useful for autologous cell therapy. Kidney Int. (2018) 93:1452–64. doi: 10.1016/j.kint.2018.01.021
98. Pappritz K, Savvatis K, Miteva K, Kerim B, Dong F, Fechner H, et al. Immunomodulation by adoptive regulatory T-cell transfer improves Coxsackievirus B3-induced myocarditis. FASEB J. (2018) 32:j201701408R. doi: 10.1096/fj.201701408R
99. Todo S, Yamashita K, Goto R, Zaitsu M, Nagatsu A, Oura T, et al. A pilot study of operational tolerance with a regulatory T-cell-based cell therapy in living donor liver transplantation. Hepatology. (2016) 64:632–43. doi: 10.1002/hep.28459
100. Bluestone JA, Buckner JH, Fitch M, Gitelman SE, Gupta S, Hellerstein MK, et al. Type 1 diabetes immunotherapy using polyclonal regulatory T cells. Sci Transl Med. (2015) 7:315ra189. doi: 10.1126/scitranslmed.aad4134
102. Ward NC, Yu A, Moro A, Ban Y, Chen X, Hsiung S, et al. IL-2/CD25: a long-acting fusion protein that promotes immune tolerance by selectively targeting the IL-2 receptor on regulatory T cells. J Immunol. (2018) 201:2579–92. doi: 10.4049/jimmunol.1800907
103. Matsuoka K, Koreth J, Kim HT, Bascug G, McDonough S, Kawano Y, et al. Low-dose interleukin-2 therapy restores regulatory T cell homeostasis in patients with chronic graft-versus-host disease. Sci Transl Med. (2013) 5:179ra143. doi: 10.1126/scitranslmed.3005265
104. Whitehouse G, Gray E, Mastoridis S, Merritt E, Kodela E, Yang JHM, et al. IL-2 therapy restores regulatory T-cell dysfunction induced by calcineurin inhibitors. Proc Natl Acad Sci USA. (2017) 114:7083–8. doi: 10.1073/pnas.1620835114
105. Ratnasothy K, Jacob J, Tung S, Boardman D, Lechler RI, Sanchez Fueyo A, et al. IL-2 therapy preferentially expands adoptively transferred donor-specific Tregs improving skin allograft survival. Am J Transplant. (2019) 19:2092–100. doi: 10.1111/ajt.15306
106. Koreth J, Kim HT, Jones KT, Lange PB, Reynolds CG, Chammas MJ, et al. Efficacy, durability, and response predictors of low-dose interleukin-2 therapy for chronic graft-versus-host disease. Blood. (2016) 128:130–7. doi: 10.1182/blood-2016-02-702852
107. Liberal R, Grant CR, Yuksel M, Graham J, Kalbasi A, Ma Y, et al. Regulatory T-cell conditioning endows activated effector T cells with suppressor function in autoimmune hepatitis/autoimmune sclerosing cholangitis. Hepatology. (2017) 66:1570–84. doi: 10.1002/hep.29307
108. Lim TY, Martinez-Llordella M, Kodela E, Gray E, Heneghan MA, Sanchez-Fueyo A. Low-dose interleukin-2 for refractory autoimmune hepatitis. Hepatology. (2018) 68:1649–52. doi: 10.1002/hep.30059
109. Whangbo JS, Kim HT, Nikiforow S, Koreth J, Alho AC, Falahee B, et al. Functional analysis of clinical response to low-dose IL-2 in patients with refractory chronic graft-versus-host disease. Blood Adv. (2019) 3:984–94. doi: 10.1182/bloodadvances.2018027474
110. Veerapathran A, Pidala J, Beato F, Yu XZ, Anasetti C. Ex vivo expansion of human Tregs specific for alloantigens presented directly or indirectly. Blood. (2011) 118:5671–80. doi: 10.1182/blood-2011-02-337097
111. Lee K, Nguyen V, Lee KM, Kang SM, Tang Q. Attenuation of donor-reactive T cells allows effective control of allograft rejection using regulatory T cell therapy. Am J Transplant. (2014) 14:27–38. doi: 10.1111/ajt.12509
112. Ellebrecht CT, Bhoj VG, Nace A, Choi EJ, Mao X, Cho MJ, et al. Reengineering chimeric antigen receptor T cells for targeted therapy of autoimmune disease. Science. (2016) 353:179–84. doi: 10.1126/science.aaf6756
113. MacDonald KG, Hoeppli RE, Huang Q, Gillies J, Luciani DS, Orban PC, et al. Alloantigen-specific regulatory T cells generated with a chimeric antigen receptor. J Clin Invest. (2016) 126:1413–24. doi: 10.1172/JCI82771
114. Boardman DA, Philippeos C, Fruhwirth GO, Ibrahim MA, Hannen RF, Cooper D, et al. Expression of a chimeric antigen receptor specific for donor HLA class I enhances the potency of human regulatory T cells in preventing human skin transplant rejection. Am J Transplant. (2017) 17:931–43. doi: 10.1111/ajt.14185
115. Noyan F, Zimmermann K, Hardtke-Wolenski M, Knoefel A, Schulde E, Geffers R, et al. Prevention of allograft rejection by use of regulatory T cells with an MHC-specific chimeric antigen receptor. Am J Transplant. (2017) 17:917–30. doi: 10.1111/ajt.14175
116. Maldonado RA, LaMothe RA, Ferrari JD, Zhang AH, Rossi RJ, Kolte PN, et al. Polymeric synthetic nanoparticles for the induction of antigen-specific immunological tolerance. Proc Natl Acad Sci USA. (2015) 112:E156–65. doi: 10.1073/pnas.1408686111
117. Kishimoto TK, Ferrari JD, LaMothe RA, Kolte PN, Griset AP, O’Neil C, et al. Improving the efficacy and safety of biologic drugs with tolerogenic nanoparticles. Nat Nanotechnol. (2016) 11:890–9. doi: 10.1038/nnano.2016.135
118. LaMothe RA, Kolte PN, Vo T, Ferrari JD, Gelsinger TC, Wong J, et al. Tolerogenic nanoparticles induce antigen-specific regulatory T cells and provide therapeutic efficacy and transferrable tolerance against experimental autoimmune encephalomyelitis. Front Immunol. (2018) 9:281. doi: 10.3389/fimmu.2018.00281
120. Dominguez-Villar M, Baecher-Allan CM, Hafler DA. Identification of T helper type 1-like, Foxp3+ regulatory T cells in human autoimmune disease. Nat Med. (2011) 17:673–5. doi: 10.1038/nm.2389
122. Okada M, Kanamori M, Someya K, Nakatsukasa H, Yoshimura A. Stabilization of Foxp3 expression by CRISPR-dCas9-based epigenome editing in mouse primary T cells. Epigenetics Chromatin. (2017) 10:24. doi: 10.1186/s13072-017-0129-1
123. Wagner DL, Amini L, Wendering DJ, Burkhardt LM, Akyuz L, Reinke P, et al. High prevalence of Streptococcus pyogenes Cas9-reactive T cells within the adult human population. Nat Med. (2018). doi: 10.1038/s41591-018-0204-6
124. Deng S, Moore DJ, Huang X, Lian MM, Mohiuddin M, Velededeoglu E, et al. Cutting edge: transplant tolerance induced by anti-CD45RB requires B lymphocytes. J Immunol. (2007) 178:6028–32. doi: 10.4049/jimmunol.178.10.6028
125. Lee KM, Kim JI, Stott R, Soohoo J, O’Connor MR, Yeh H, et al. Anti-CD45RB/anti-TIM-1-induced tolerance requires regulatory B cells. Am J Transplant. (2012) 12:2072–8. doi: 10.1111/j.1600-6143.2012.04055.x
126. Lee KM, Stott RT, Zhao G, SooHoo J, Xiong W, Lian MM, et al. TGF-beta-producing regulatory B cells induce regulatory T cells and promote transplantation tolerance. Eur J Immunol. (2014) 44:1728–36. doi: 10.1002/eji.201344062
128. Le Guen V, Judor JP, Boeffard F, Gauttier V, Ferry N, Soulillou JP, et al. Alloantigen gene transfer to hepatocytes promotes tolerance to pancreatic islet graft by inducing CD8(+) regulatory T cells. J Hepatol. (2017) 66:765–77. doi: 10.1016/j.jhep.2016.11.019